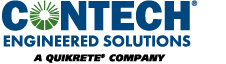
From California to Maryland, high-rate biofiltration continues to gain acceptance within the regulatory and engineering community as an acceptable alternative to traditional bioretention. These systems provide high treatment levels in a compact size (see Figure 1), making biofiltration feasible on sites where traditional bioretention is not possible. They have earned numerous agency approvals for meeting water-quality standards for post-construction best-management practices (BMPs). In addition, they benefit from being smaller in size, which saves valuable land space and reduces long-term maintenance costs compared to traditional bioretention.
Bioretention has been the principal form of Low-Impact Development (LID) used to slow, treat, retain and infiltrate stormwater runoff, mimicking a site’s natural, pre-development hydrology. Both traditional bioretention and high-rate biofiltration are pollution-control mechanisms that incorporate physical, chemical and biological (living) processes via vegetation to capture, immobilize and treat harmful pollutants from stormwater runoff. Other terms that describe this process include rain gardens, vegetated filters, bioswales, biofilters and tree-box filters.
High-rate biofiltration differs from traditional bioretention in that it has been optimized for high volume/flow treatment and high pollutant removal. Traditional bioretention footprints with typical design flow rates of 1-12 inches per hour can occupy up to 10% of the contributing impervious drainage area. In addition, the individual components of traditional bioretention (mulch, soil, underdrain and plants) are designed by the engineer, and sourced and installed by the contractor.
In contrast, high-rate biofiltration systems contain an engineered high-flow biofiltration media with flow rates up to 175” per hour (depending on the jurisdiction). In addition, these systems are packaged and delivered with all the components necessary for system performance: mulch, media, vegetation and underdrain.
Figure 1. An example of a high-rate biofiltration system.
It should be noted that not all systems marketed as high-rate biofiltration include vegetation. “Bio” implies that living organisms are a component of the treatment processes that work in conjunction with other pollutant-removal mechanisms. Without plants as a critical component of the synergistic community of living organisms in these systems, biofilters may not be as effective over the long term and may not meet the LID requirements of local jurisdictions. Therefore, it’s crucial to verify that any high-rate biofiltration system you are considering to specify includes vegetation.
To understand the physical and biological processes in a high-rate biofiltration system, it’s necessary to look at how each component of the system (see Figure 2) contributes to pollutant removal and flow management.
Figure 2. A typical high-rate biofiltration system includes multiple components. Mulch
Often seen as just an aesthetic feature, mulch plays an essential role within the system. It provides physical filtration and acts as a pretreatment and anti-scour layer for the media. In addition, the mulch layer protects biofiltration media from occlusion by capturing sediment loads and debris. Properly maintaining the mulch layer will extend the service life of the bioretention soil media indefinitely in both conventional or high-rate systems. In addition, mulch provides benefits beyond pretreatment. Since the mulch is made from organic material, as it biodegrades, it releases new organic material into the media, which helps regenerate the media and sustain the biological processes taking place within the media. The mulch also provides water retention for the system’s vegetation.
The mulch is the only component of the system that needs to be replaced regularly, usually one to two times per year.
Recycled yard waste from public municipalities or recycled wood scraps such as pallets should be avoided due to inconsistencies in source and potential for unwanted trash or chemical additives in the mulch.
The mulch should not float, as floating mulch could be transported out of the system, allowing sediment fines to accumulate on the media layer. Denser plant covers also can reduce mulch flotation. Mulch with higher moisture content and, therefore, greater density also can minimize floatation. Bark nuggets and wood chips should be avoided due to their lighter density and texture that lends itself to flotation.
The mulch should not be oversized, as large voids will not capture sediment. On the other hand, if the mulch is too finely ground, it will not provide the proper flow properties to allow the water to enter the media layer. Finally, the mulch should not have any amendments added, such as fertilizers or pesticides, as these represent elements that are often the focus of pollutant removal.
Inorganic mulches commonly available include rock mulch, landscape fabric, plastic sheeting and rubber mulch from recycled tires. While this is a preferred method for some landscapes, since they do not degrade as quickly and require less maintenance, inorganic mulches are not optimal choices for biofiltration practices as they do not maintain or improve media quality through time.
To ensure the selection of quality mulch for your high-flow biofiltration system, it’s a good rule of thumb to ensure it has the Mulch & Soil Council Certification emblem on the bag (see Figure 3). Mulch products with this certification must pass a rigorous screening and are periodically audited to ensure the products meet Council standards. The certification ensures the product label is accurate, all ingredients are listed and product claims have been verified. A Mulch & Soil Certification also ensures the mulch contains no chromated copper arsenate, a wood preservative found in recycled pilings, utility poles and highway structures. If mulch certified by the Mulch & Soil Council is not available, it should be free from waste wood material, harmful chemicals or inorganic dyes. If mulch is being used in a nutrient-sensitive area, a leachate analysis will ensure the mulch does not contribute to nutrient leachate from the practice.
Figure 3. The Mulch & Soil Council Certification emblem.
After the mulch layer, water flows through a media layer, which filters fine pollutants and nutrients through physical and chemical processes. The composition of high-flow media differs by manufacturer but typically contains a proprietary mixture of a specified gradation of washed aggregate and organic material that’s homogeneously blended.
The media composition allows for adsorption of pollutants such as phosphorus in the form of phosphates or dissolved metals that are chemically attracted to the soil components and removed via reactive filtration.
Components of engineered bioretention media support both inter- and intra-storm treatment processes. Aggregates in the engineered media increase and maintain flow rate and provide aeration to vegetative roots. Organic matter retains moisture, provides carbon to the microbial community, supports vegetative growth and enhances pollutant removal. These components support inert and reactive filtration during storm events as well as the biological transformations and sequestration that occur between storm events.
If the mulch layer is maintained correctly, the high-flow media will not need to be replaced. The chemical and biological processes between the media and the vegetation will continually regenerate the media.
Most of the biological processes for which biofilters are named occur underground in the rhizosphere. The rhizosphere is the habitat where the plant root system interacts with the soil environment via root secretions and soil microorganisms. Millions of diverse microorganisms exist in the rhizosphere and exist to this magnitude only because of the plant. For example, there are 10x more microorganisms in the rhizosphere than outside of it.
But why are microorganisms important? First, microorganisms degrade and transfer pollutants into less-toxic forms, which the plant then can uptake and sequester through carbon and nutrient assimilation. Second, microorganisms alter the soil chemistry in the rhizosphere, which enhances pollutant-removal efficiency. Third, plants increase organic matter in the soil through the decomposition of biomass, including the roots themselves, known as cell sloughing, which provides a carbon source to the microorganisms in the media. Additionally, mycorrhizae fungi create a symbiotic relationship with plant roots whereby plant roots excrete sugars for the fungi, while the fungi provide “pollutants” to the plants in the form of nutrients for further biomass production. Finally, mycorrhizae fungi increase the surface area of plant roots, which ultimately enhances the absorption of phosphorus, nitrogen and metals, which are all macro and micro plant nutrients vital for plant growth and reproduction.
Sustainability is characterized by the system’s ability to maintain high pollutant removal and design hydraulic flow rates through time. Plants help maintain the permeability of the media through their expanding root systems. Roots shrink and swell during wetting and drying cycles, keeping the filtration media aerated. Plant roots continuously penetrate filter media as the plant grows, and the roots themselves die and regrow, forming micro-channels. This process prevents media compaction and increases porosity, ultimately maintaining aeration and infiltration rates. In addition, plant roots and associated microbiological growth provide exudates that build and maintain soil structure. Plants also enhance volume reduction through evapotranspiration.
Plants also play a vital role in the long-term sustainability of the filtration media. As the filter media captures and immobilizes pollutants, those pollutants are decomposed, volatilized and incorporated into the biomass of the system’s micro/macro fauna and flora. In addition, the cycling effect in which pollutants enter the system, are biodegraded by the microorganisms in the soil, and then are taken up into the vegetation helps regenerate the media for the next rain event, as there are more reaction sites available for new pollutants that enter the system.
Systems without vegetation do not have the same ability to transform and sequester pollutants in the biomass. They also have less capacity to maintain the hydraulic properties of the filter media through time. As a result, they may require regular media replacement.
Who specifies the vegetation varies by application and may include the engineer, the owner or a landscape architect. Regardless of who specifies the plant palate, specific guidelines need to be followed. Most manufacturers of high-rate biofiltration systems provide plant lists organized by hardiness zone to guide plant selection. The plants selected should be readily available within the community where the system is located. Selected plants should tolerate low-moisture soils, as the media is designed to drain down between storm events. In arid climates or where there are extended seasonal dry periods, irrigation should be provided to maintain plant vitality. Typically, small grasses, shrubs or small ornamental trees with fibrous root systems are selected. Larger trees can be accommodated by constructing a custom vault with openings that allow for root penetration into the surrounding soils.
All high-rate biofiltration systems are designed to drain down via infiltration into the surrounding soils or through an underdrain. This drain down is needed to ensure there’s no standing water in the media for long periods. If the surrounding soil can’t accept the runoff, an underdrain is needed to allow the water to drain down. Proper sizing of the underdrain is vital due to the high flow rates the system will encounter.
Many high-rate biofiltration systems come pre-packaged as a complete system in a concrete containment structure. These structures can be designed with or without a top slab and tree grate (see Figure 4).
Figure 4. An open-top high-rate biofiltration system in Carlsbad, Calif.
The structure’s purpose is to house the internal components and enable fast installation. During installation, the structure containing the underdrain and media is set in place and backfilled, with the installation of the plant and mulch occurring after the site is stabilized.
Large systems (generally greater than 300 square feet) can be designed without a containment structure, as the structure would be too large to manufacture and ship (see Figure 5). Doing so adds complexity to the installation process, but it provides size and maintenance advantages compared to large, regional systems that use traditional bioretention.
Figure 5. The Whispering Firs Stormwater Park in Silverdale, Wash., uses highrate biofiltration to treat runoff from 115 acres of development.
Due to the high media flow rates (100-175 inches per hour), high-rate biofiltration has a much smaller footprint than traditional biofiltration, typically less than 0.5% of the impervious drainage area (see Table 1). The smaller footprint results in more usable land, which can be advantageous on sites where the cost of land is expensive or on tight, highly developed sites such as urban development projects, commercial parking lots, residential streets and streetscapes. The small footprint also reduces installation and lifecycle costs vs. traditional bioretention, as there’s less square footage to maintain. However, it’s important to note that a smaller bioretention system footprint doesn’t necessarily mean the total amount of green space on a site will be reduced. For example, the land area saved by specifying high-rate biofiltration can be used for conventional landscaping or for dedicated recreation or habitat areas where stormwater pollutant accumulation and prolonged inundation during storm events could be problematic.
Table 1. Benefits of High-Rate Biofiltration
Another benefit of high-rate biofiltration systems is that they are manufactured in a controlled environment, with all the components sourced by the manufacturer to ensure the final media product performs per design specifications. This differs from traditional bioretention, where the contractor sources and installs each component separately, and the media is blended onsite. Media blending methods vary, most often depending on the amount of media to be mixed and the equipment available. Like two different cooks can use the same ingredients to create two different meals, different blending methods can have different final results, even with the same components. To reliably achieve consistent media properties, specific standards of practice for media production must be followed in a controlled environment.
Since its inception in 1996, the International Stormwater BMP Database (www.bmpdatabase.org) has been archiving performance, design and cost data for stormwater treatment BMPs in a publicly accessible database. At this point, more than 600 BMP studies have been added, providing a wealth of information to regulators, engineers, academics and others seeking to understand and improve the state of the art of stormwater management. At the end of 2020, a new report was published that summarizes data made available in the December 2019 release of the database.
The new report includes six studies of “High-Rate Biofiltration.” Comparing the results for high-rate biofiltration to traditional bioretention results shows that high-rate biofiltration performs similarly or better for most pollutants compared to traditional bioretention. Median effluent concentrations (see Table 2) for total suspended solids, total phosphorus, total copper and dissolved copper are significantly lower for high-rate biofiltration (HRBF) vs. traditional bioretention (BR), as indicated by non-overlapping 95th percentile confidence intervals. Phosphorus performance is the most divergent parameter, with traditional bioretention showing a significant increase from influent to effluent, and high-rate biofiltration showing a significant reduction. Traditional bioretention median total zinc effluent is significantly lower than for high-rate biofiltration; however, both BMPs provide significant reductions.
Table 2. Bioretention and High-Rate Biofiltration Performance from the 2020 Summary Statistics Report by the International Stormwater BMP Database
Source: The Water Research Foundation, 2020. International Stormwater BMP Database: 2020 Summary Statistics. Project Number 4968. https://www.waterrf.org/system/files/resource/2020-11/DRPT-4968_0.pdf
High-rate biofiltration continues to gain acceptance within the regulatory and engineering community as an acceptable alternative to traditional bioretention. Its high flows, small footprint and pollutant-removal capabilities allow for a low-impact development design strategy on sites where land cost is expensive or on tight, highly developed sites with limited land space for traditional bioretention.
Alex MacLeod, P.E., Mindy Hills, CPSWQ, David Corr and Vaikko Allen are with Contech Engineered Solutions; email [email protected], [email protected], [email protected] and [email protected] respectively.
Online quiz for this article is not active and
PDH credit is no longer available.
This article is being maintained for informational purposes only.