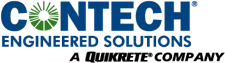
A constant challenge among our nation’s infrastructure managers and their engineering partners is to determine the most cost-effective, long-lasting bridge structure that will fit the many constraints of today’s bridge replacement project. Many deficient bridges across America can be found on rural, low-volume roads that are ideally suited for prefabricated steel truss bridges.
These constraints may include environmentally sensitive stream crossings, or expensive railroad grade separation projects, coalescing public input and support, or projects where the need for speed is imperative. Many of these raise the cost of the project without any tangible benefit. A long, clear span is proven to solve many of these problems. By virtue of an impressive span-to-weight ratio, steel truss bridges can eliminate piers, improve hydraulics and reduce installation costs where other bridge types cannot. Further, an often-repeated reason for specifying steel truss bridges banks on the fact that people like them; they enhance the landscape and create a signature impression to the traveling public.
Steel truss bridges continue to be one of the most effective and economical bridge types selected by developers and local infrastructure managers for single spans of more than 100 feet. For rural highways over streams or railroads, a steel truss bridge often is the best all-around solution. Because of the arrangement of floor framing members connecting into truss elements, the bridge’s structural depth can be minimized as compared to multiline, under-deck girder systems. This arrangement is characterized by below-deck, floor beam members spanning the shorter dimension across the bridge width to primary truss girders outside of the bridge’s roadway or deck. Because these floor beams span the shorter direction, they are much shallower than would be needed to span the entire gap between abutments. The truss girders that span the gap can be sizable, deep truss members, but because they are beyond the roadway, they project above the roadway allowing for a minimized depth from the roadway surface to the underside of the lowest framing member. This saves costs due to less embankment material, less pavement construction, less impacts to nearby property owners and simpler permitting.
Site conditions and limitations usually drive the search for the shallowest superstructure depth. For rural roads or low-volume city streets where a single span is desired, or where the number of pier structures should be minimized, a steel truss span or spans should be evaluated as a very feasible alternative.
In addition to the shallow superstructure depth a truss bridge affords, several other features or cost considerations enter the evaluation. These primarily involve the ability to deliver and erect the bridge members. Because a steel truss bridge can be prefabricated into deliverable segments and spliced together at the job site, it allows for smaller shipments, more simple delivery and less costly trucking permits. Once spliced together, it can also mean much lighter crane lifts. Usually these crane lifts can be made from the approach roadway, again limiting the impacted footprint of the project. For comparison, a single precast box or I-girder may weigh up to three times as much as an equivalent length truss member. This requires a heavier crane for a longer duration and likely will need a crane position and staging area adjacent to the bridge span. For stream crossings this can mean a causeway in the stream; for railroad spans this can mean higher insurance costs for working in the railroad’s right-of-way with special flagging controls for having equipment and workers near the tracks.
Prefabricated steel truss bridges can be fabricated in many different styles, finishes and configurations. They can be utilitarian in their appearance or they can be designed to enhance a developed area or become an aesthetic landmark for users of the facility. There are many possibilities. It is a matter of deciding what is important and what you envision your project to be.
The truss style is basically the shape seen in the elevation view of the bridge. Generally speaking, the geometry of today’s prefabricated truss bridges vary in two primary ways: the shape of the top chord and the arrangement of the web members. A top chord may be curved in an arc, it may be flat, or it may be segmented to form a polygon or camel-back. They each have different visual impacts. The web members, diagonals and verticals, generally are arranged in either a Warren Truss configuration or a Pratt Truss configuration (see Figures 1 and 2). These web members are spaced regularly across the elevation in panels that usually range from 10 to 18 feet. No matter the style chosen, the loads of the bridge flow generally in the same manner from the deck, into the floor framing and truss, resolving into force reactions on to the bearings.
The common span range for prefabricated steel truss bridges is 75 to 250 feet and is defined by the plan dimension between the centerline of each bearing at the forward and rear abutments. This dimension sometimes is confused with “bridge length.” In general, overall bridge length can be thought of as the gap that needs to be crossed or filled; or the opening between the abutment’s backwalls. The difference between span and length requires an understanding of the end-ofbridge details that describe the extension of the structure beyond its bearing points as it reaches to the substructure (Figure 3). This is determined during the abutment design and is influenced by the requirements of any called-for expansion joint.
Truss bridges may be oriented in a skewed manner, meaning that the beginning station of the left-side truss is not the same as the right-side truss, as in a staggered configuration. Some flexibility exists in arranging the floor framing of a skewed truss span as it may follow the skewed alignment or it may be arranged orthogonally to the trusses. Each of these attributes is inherent to steel and greatly enhances the flexibility of the bridge to conform to the site geometry while maintaining an economic arrangement.
Prefabricated steel truss bridges vary in width from one lane up to three lanes, depending on lane width and shoulders, but have a practical width limit of about 50 feet. Bridge width is defined as the transverse dimension between railings, parapets or curbs and needs to be sufficient to accommodate the lanes and shoulders of the approaching roadway. Sidewalks also may be considered part of the bridge width, as shown in the Through and Half-Through Truss sections in Figures 4 and 5. They can be accommodated either between the trusses on an elevated curb, or outside the trusses using cantilevered framing. If an internal sidewalk is desired, the specifier should give some consideration to the separation of traffic and pedestrians either in the form of a barrier or an unmountable curb, depending on the facility and the anticipated amount of pedestrian traffic.
Another factor in designing the transverse section of a truss bridge is determining whether the bridge will require portal and sway bracing. The Through Truss section shown in Figure 4 typically is utilized on longer-span bridges to brace the truss top chords to allow them to achieve higher capacities without buckling. The combination of span, bridge width, skew and specified loads will determine whether these members are necessary and should be determined early on in preliminary engineering. Once identified, a vertical clearance above the roadway will need to be specified for these overhead bracing members.
After defining the span and width, the bridge’s floor or deck construction becomes the next key attribute a specifier must decide upon. The selection of a floor or deck construction has the largest effect on the remaining details and the costs to construct the bridge. Here a bridge “floor” is defined as one made up of concrete, timber or corrugated steel planks. Bridge flooring usually is filled or topped with a driving surface. A bridge “deck” will mean reinforced concrete deck slab. Varieties of floor or deck options exist and have a large effect on edge details, railing support, durability and longevity. Several questions that should be answered before specifying a floor or deck construction are:
Longevity of the bridge and the expected maintenance activities are important concerns for a bridge owner. As such, steel bridges require surface protection against aggressive environments. Like other steel bridges, prefabricated bridges may be manufactured using weathering steel (Photo 1), protective hot-dip galvanized steel (Photo 2) or a multi-coat paint system (Photo 3). Each has its merits and limitations and should be specified carefully.
Weathering steel has a rustic, natural look that lends itself to scenic settings. Because it has fewer operations to perform in the manufacturing process, it generally is considered the least expensive. Its primary limitation is that of proximity to open water, but also it sometimes is difficult to procure on a short schedule.
Hot-dip galvanizing offers superior corrosion protection and is specified the most. Galvanizing has a silverto- gray appearance and blends into a background scene very well. Some manufacturers offer a 35-year warranty through their galvanizer that ensures a period to “first maintenance” (See references for additional learning resources).
Specifying a paint system also is a viable and often used protection method. It provides quality protection with an appearance that otherwise cannot be achieved. Manufacturers should be AISC endorsed and have extensive experience painting bridge structures to ensure a quality application that will last. Painted structures usually are used where aesthetics are important and the bridge is envisioned as a focal point.
Corrosion protection systems may even be combined in different ways, e.g. painting over galvanizing, or partial painting of weathering steel areas or even the combining of a galvanized floor framing with weathering steel truss members. The selection of combined systems should be made to enhance a particular advantage from one of these individual systems.
When you consider specifying a modern steel truss for your project, it may seem like answers to questions are a little more difficult to come by. How shallow is the floor beam? What kind of railing construction is available? Should I consider a curbed section? How many trucks will it take to deliver? How much do the trusses weigh? What cost range should I assume for my budget? How are these bridges erected?
The initial scoping process typically involves the bridge specifier contacting an AISC-certified manufacturer to help scope key dimensions, common details and options. From this initial conversation, an engineer with the manufacturer can begin to develop preliminary member sizes and load reactions for the substructure design. Experienced manufacturers will go an extra step by developing framing plans and transverse sections at this early stage to depict the project schematically. If the project will be public, a manufacturer may supply the owner agency with CAD drawings for their use in completing the bid documents. Model specifications also are available for editing and commonly are supplied at this stage.
Many times bridge steel needs to be purchased ahead of final approval because of critical lead times related to mill rolling dates. Because of this, accurate specifying of the bridge in the initial contract documents is extremely important. If these documents are vague or incomplete, the prefabricator must sort out the owner’s intent with questions, requests for information (RFIs) or interpretations, all which slow the design and procurement steps. To ensure a quality process, the specifications also should require the prefabricator to be AISC-certified as a Major Bridge Fabricator, and have the necessary experience and proven ability to deliver the bridge that is specified. These specifications should be demonstrated in a qualifications package supplied by the prefabricator.
The formal design process usually is initiated after a contract award is made. Design criteria will follow AASHTO’s design specifications, either Standard Specifications for Design of Highway Bridges, 17th Edition, or LRFD Bridge Design Specifications, 6th Edition. Additionally, state manuals governing DOT requirements must be considered. In these documents are hundreds of requirements that pertain to the bridge design. It is very important to select a bridge prefabricator that has deep knowledge of these design codes and the experience to properly interpret the provisions. This helps prevent delays and ensures a safe and complete design. Installation drawings are prepared and should be thought of as an extension of the contract construction drawings. A project detailer further develops drawings that depict the fabricated members in traditional shop drawings. This dual plan set guides the contractor to complete the field work and it guides the shop production of each steel member. A typical plan set for installation plans and shop drawings will contain anywhere from 15 to 40 sheets, depending on the size, skew, symmetry and features of the bridge. The calculation and drawing package is then sealed by a Professional Engineer licensed to practice in the state where the bridge is located and is submitted to the customer for approval.
Two tools that are enabling bridge prefabricators to increase productivity and quality are Computer Numerically Controlled (CNC) equipment and Bridge Information Modeling (BrIM) software.
Bridge structural components that are processed on CNC equipment are first programmed by software that defines the operations, tooling and quantities for each piece. Bridge Information Modeling (BrIM) allows for a seamless exchange of design data to the machines. Specifying that steel be processed with CNC equipment and programmed with a complete 3D Bridge Information Model will shorten production times and increase the quality of the fabricated bridge.
After steel is received from the mills, it is processed in steel fabricating shops using equipment and manpower to drill, cut and curve the components, then weld or bolt the shippable assemblies together. Other items for construction that might be ordered for the project, such as timber floor, guardrail, bearings and light-gage concrete forms, are procured and readied for shipping to the site.
Quality control measures are used to verify that pieces are fabricated accurately and assembled according to specified standards. These standards should include the American Institute of Steel Construction (AISC) Steel Construction Manual, AASHTO/NSBA’s S2.1 Steel Bridge Fabrication Guide Specifications, the American Welding Society (AWS) D1.5 Bridge Welding Code and the Research Council on Structural Connections (RCSC) Specifications for Structural Joints Using A325 or A490 Bolts.
Depending on the size of the bridge, members will be shop assembled into erectable units to speed construction. The size and weight of these units will be shown in the construction drawings to assist the contractor in planning the installation. These units, along with other individual components, will arrive on the site on a series of trucks. The components are marked according to the drawings which are then used to guide the contractor’s installation. Technical assistance from the prefabricator’s staff, who is experienced in erecting steel bridges, is recommended during determination of the specifications to ensure a smooth installation.
The history of steel truss bridges dates back to the 1800s, and prefabricated steel truss bridges, back to the 1930s when modular systems were developed for military purposes. A modern steel truss bridge has proven time and time again to be a feasible, attractive and economic solution for bridge sites that have difficult engineering challenges. Specifying a modern steel truss bridge can be simplified with the help of a bridge manufacturer that has the resources and experience to lead the way.
Dennis L. Gonano, P.E.,is the Director of engineering and department manager for U.S. Bridge in Cambridge, Ohio.
James G. Bauer, B.S./M.B.A.,is Product manager for Truss Bridge Products for Contech Engineered Solutions. His nine years at Contech has included roles in sales, training and precast concrete bridge product management.
Online quiz for this article is not active and
PDH credit is no longer available.
This article is being maintained for informational purposes only.