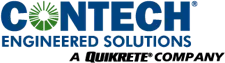
When properly designed, an Articulated Concrete Block, also called ACB, low-water crossing can provide a hydraulically-robust load-bearing system while also facilitating fast-paced design and construction schedules. This article focuses on the three critical design elements of a successful low-water crossing project: design and construction of the load-bearing subgrade, hydraulic stability of the crossing and its surroundings, and the use of articulated concrete block as a flexible roadway surface.
There are thousands of roadways providing back-country access across the North American continent where existing drainage structures, such as bridges or culverts, are in need of replacement. In addition, roads continually are being built to provide access to new sites for recreational development and commercial expansion for which drainage structures are required. Access roads in need of drainage remediation both new and old often are located in rural areas with relatively low traffic volume making low-water crossings a viable and cost-effective option to consider.
The Natural Resources Conservation Service (NRCS) in its "Conservation Practice Standard for Stream Crossings" (References 5), defines a low-water crossing as "a stabilized area or structure constructed across a stream to provide a travel way for people, livestock, equipment or vehicles". This article focuses on equipment or vehicular loading as it relates to the use of articulated concrete block mattresses in low-water crossing designs. These designs consist of a properly-designed subgrade to support the anticipated loads, and articulated concrete block mattresses installed for erosion control and sized for hydraulic stability. The articulated concrete block mattresses serve a dual purpose of erosion control armoring and as a flexible roadway surface.
The first step with any vehicular loading design is to determine the load to which the roadway will be subjected. This will depend on what kind of vehicle and associated wheel loads that will be traveling on the system, as well as the frequency of use. Roadway section design is a well established civil engineering practice which enables loading characteristics and standard loading sections to be applied to low-water crossings. The most common vehicular loading used for low-water crossings design is AASHTO HS 20-44.
As with most vehicular load or bearing capacity designs, the strength of site soils defines the thickness of the recommended section beneath the armoring or traveling surface layer. Standard AASHTO design tables should be used whenever practical to determine the section beneath the articulated concrete block (References 1).
AASHTO design criteria are sometimes difficult to implement for a project due to remote site locations, availability of construction materials and the like. In these cases, design engineers must understand that the intent is to assure that the section is firm and unyielding when subjected to the design load and make their decisions accordingly, exercising engineering judgment. Examples of such non-standard loadings would be all-terrain vehicle trails or logging/mining roads which are subjected to significantly more or less than the standard HS 20-44 loading. In low-water crossing applications, the articulated concrete block veneer is subjected to crushing only and cannot be assumed to benefit or improve the site soils to assist with the design load.
Designers can estimate soil bearing capacities based on a myriad of soil testing, inspection and applied engineering judgment. The NRCS has three basic classifications for site soils based on effective stress analysis: well to moderately drained soil, somewhat poorly drained soil and poorly drained soil.
Another method for determining the roadway section beneath the articulated concrete block system is the California Bearing Ratio (CBR) technique to determine soil strength in the field. For example, as seen in Figure 3 and Table 1, section thicknesses beneath the articulated concrete block system can vary with respect to the CBR value of the site soils. These values can be reduced with the use of a reinforcing material such as a high-strength geotextile or geogrid material, reducing the overall section thickness and required excavation.
CBR | 1 | 2 | 3 | 4 | 5 |
UNREINFORCED STONE THICKNESS, H (IN) |
61 | 31 | 20 | 15 | 11 |
REINFORCED STONE THICKNESS, H (IN)* |
36 | 16 | 8 | 8 | 8 |
Note: This section is developed assuming 1,000 passes of HET military loading *2 layers of TYPE 1 GEOGRID at subgrade and middle of drainage box. |
In Shannon County, Missouri, an ArmorFlex® mat was installed at the low-water crossing of County Road 401 over Mahan Creek near the Ozarks. The original crossing consisted only of a natural stream gravel bed, meaning continuous road repairs after flooding and safety concerns for local residents. An articulated concrete block mattress solution allowed for improved stability at the crossing for local residents, while not impeding the passage of stream animals. | |
Photo 1: Installation of interlocking mats to form a mattress in the low-water ford.
![]() Photo 2: Completed installation provides a stable low-water crossing.
![]() |
Designers should implement best management practices (BMPs) when working around streams. The first BMP emphasizes minimal environmental impact. In proposed low-water crossing design, the most favorable or optimal alignment is having the structure oriented 90o to the centerline of the channel (perpendicular to the flow direction). This exposes the structure to the least amount of flowing water. Designers should strive to hold this favorable design requirement when practical as it relates to low impact development (LID) construction and intrusion. However, skewed crossings are commonly encountered, especially when replacing a pre-existing structure.
The general layout for a low-water crossing is commonly predetermined as the approach width and alignment are somewhat dictated by the existing out-of-stream roadway dimensions and grade. Another BMP involves the general location of the structure within the stream. Unlike existing stream crossings where the plan form has been determined previously, new low-water crossings such as expanded federal and state park roads, fire trails, commercial logging trails or recreational roads, can utilize BMPs.
When designing a low-water crossing, the structure should conform to the site's profile as much as possible. A designer should emphasize details such as keeping the top elevation of the driving surface level with the surrounding natural bed, thus minimizing potential obstacles. Any significant changes to the general bed form can change the local flow regime, having an undesirable effect on aquatic organisms. Following these BMPs will assist the designer to integrate the low-water crossing structure harmoniously into the surrounding environment while addressing the remaining critical questions:
Typical stabilized and armored low-water crossing dimensions have general travel lane guidelines, dictated by the approaching roadway lane widths. Surrounding topography and vegetative growth also can prove to be factors when determining the cross-sectional dimensions of proposed low-water crossings.
According to NRCS Code 560 (References 5), the overall exposed width of a one-lane crossing including 2-foot shoulders is 14 feet. For two-lane traffic, the minimum width including 2-foot shoulders is 20 feet. These general guidelines result in tread widths of 10 feet for one-way traffic and 16 feet for two-way traffic. When a one-lane low-water crossing design is required, it is recommended the roadway before the low-water crossing approaches be widened by 10 feet to allow for safer access into and out of the stream. The NRCS standards can be used as a basis for designers utilizing engineering judgment when facing unique site challenges outside of the recommended standards.
Low-water crossing approach dimensions are significant as they will define the overall exposed traveling length for the vehicles using the structure. The designer can choose from two basic geometric designs to define grades of the approach slopes. The first and most common is matching existing channel cross sectional dimensions (i.e. approach slopes, bed width, side slopes, etc.). The second is to employ a maximum approach grade no steeper than 4H:1V, which generally can be assumed geotechnically stable with even poorly drained, low strength soil types (References 5). The approach limits, into and out of the low-water crossing, are directly related to the design event flowing through the section (see Figure 1). As a result, this common channel hydraulic analysis can also be used to determine the size (thickness) of the articulated concrete block system used for the low-water crossings structure. When analyzing crosssectional flow areas for armoring limits, guidelines used by the United States Forest Service (References 6) define the recommended armoring as the wetted perimeter plus one foot minimum freeboard (see Figure 1).
Maintaining stream stability specifically involves addressing possible scour beneath the proposed structure or any adjacent bank erosion or excessive deposition. While scour depths can be calculated based on d50 size of the bed load and stream velocity, it is often impractical to perform an extensive scour analysis on a low-water crossing. During pre-design, the engineer should inspect upstream and downstream of the proposed low-water crossing for indicators of bankfull discharge, natural or man-made obstructions and measures of the scour depths associated to the stream. These measured depths should be incorporated into the armoring limits and terminations of the structure, which will essentially function as a cut-off wall countermeasure for scour. The completed low-water crossing should be inspected following a design event to verify that appropriate embedment depths have been realized.
![]() |
![]() |
Inherently, any attempt to manage stream stability can prove to be a challenging task. According to the U.S. Forest Service, stream stability can be defined as the capacity of a stream or channel to transport its water and sediment inputs without changing its dimensions, such as width, depth, cross-sectional area and slope (References 6). The amount of effort to effectively determine stream hydraulics can be extensive, and it is common for federal and state agencies to have classification worksheets. Stream types include perennial, intermittent and ephemeral as defined below:
Perennial: | Flowing water in well-defined channel 90% of the time |
Intermittent: | Flowing water 50% of the time |
Ephemeral: | Flowing water for short duration after severe storms in an undefined channel |
Classifying the type of stream assists the designer to exercise engineering judgment addressing how conservative to make the low-water crossing design. Stream characteristics — such as the Manning's roughness coefficient, drainage area and design discharge — also are included in the agency worksheets. As with any design, any additional information made available to the designer will allow for a more efficient and possibly less expensive project.
Determining the design discharge is crucial as this value is associated directly with the crosssectional limits of the armor and robustness of the armoring. How this design discharge is determined varies from place to place, depending on the site location and/or owner of the stream. Owners or agencies usually have drainage areas well defined within their geographic boundaries.
A good example of this is the state of Iowa, which uses the drainage area and return exceedence values to determine daily design discharge (See Figure 2). This is shown as Qe = aAb, where Qe is the design discharge in cubic feet related to an exceedence probability (e) and A is the drainage area in square miles which is either a known value or can be estimated using U.S. Geological Survey (USGS) quadrangle maps. Iowa provides additional guidance by including three regions to account for changes in topography that define the state and their corresponding values for a and b, derived from historical daily discharge data (see Figure 2). While most stream design directly relates to yearly flow exceedence (Q2 , Q100 , Q500 , etc.), deciding which design event to use to develop the overall site geometry and limits of the armoring system, is a process that has many schools of thought that vary from state to state; and occasionally within the state itself. Local design codes and standards always should be investigated.
Because scour is a primary design variable in proposed and existing crossings, it is usual practice for the armoring system, in this case articulated concrete block, to be analyzed with respect to highest velocity and shear forces resulting from design discharges. These flows commonly are associated with more frequent return intervals (Q2 , Q5). In these more common flows, tailwater effects can prove minimal or non-existent, allowing for increased velocity as the primary design parameter.
However, using these return intervals might not adequately define the physical limits in need of armoring. With regard to cross-sectional geometry, discharges with lower storm frequencies (Q50 , Q100 , Q500) dictate increased flow depths consequentially increasing the armoring limits for the approaches.
Once the overall channel hydraulics and site geometry have been addressed, designing articulated concrete block systems for low-water crossings can be divided into two separate and straight-forward design tasks.
In low-water crossing applications, articulated concrete block can be defined as an "overlay" with known hydraulic capabilities. Having the proposed/existing geometry (limits) with known hydraulics and vehicular loading scenarios addressed, articulated concrete block factor of safety analysis for hydraulic stability is employed to adequately determine the thickness and block footprint of the system. Articulated concrete block thicknesses across various applications typically range from 4.75 to 8.5 inches. A 6-inch articulated concrete block unit is a common default thickness for vehicular loading, with thicker blocks required to address more severe hydraulic forces acting on the system.
Hydraulic analysis for articulated concrete block has well-established factor of safety methodology based on full-scale testing to determine the hydraulic capability of that particular system. For the articulated concrete block products, even subtle differences in overall shape, cross section and minor block details can result in very different acceptable shear and velocity thresholds. Because of these differences, it is highly recommended designers ask for these values and test reports from the articulated concrete block producers being considered for the project. Further information on the articulated concrete block system and how it is designed, may be found in "Articulated Concrete Block Design", another technical article developed for the PDH program. (References 2).
Low-water crossings armored with articulating concrete blocks present a low-cost solution for drainage structure requirements for remote access or low-volume traffic, drainage remediation and new site development. When utilizing design best management practices and low impact development techniques, low-water crossings can be integrated harmoniously into the surrounding environment while maintaining low life-cycle maintenance costs.
As in all roadways, the most critical element is to properly evaluate and design an adequate subgrade to support the anticipated loads. Second, selecting an appropriately-sized articulated concrete block to withstand the known or estimated hydraulic forces and serve as the flexible surface of the roadway, will assure the longevity of the structure. Proper terminations and toe-ins are required to integrate the structure into the surrounding environment while protecting the edges of the system from scour and erosion.
Online quiz for this article is not active and
PDH credit is no longer available.
This article is being maintained for informational purposes only.